Summary
Geogrids are one type of the geosynthetic family. Geogrid is a material made of polypropylene and polyethylene, or a combination of these materials, that is currently widely utilized in the construction of highways, runways, railroads, earembankments, slope stabiliy, and other applications. Because of their exceptional properties, geogrid can be utilized directly to strengthen and reinforce soils.
Introduction
Soil has been used as material for construction for many years. Early man, who didn’t yet have the technology of making artificial materials, was logical to utilize the resources that were available in nature such as stone, wood, and soil to build shelters and routes for himself. As a result, soil is one of the first building materials used by people and with passing ages, no replacement has been developed, and this may have more to do with economics than anything else, because soil and stone are the most abundant resources in nature, and replacing them with artificial materials in huge quantities is not financially feasible.
However, there are various types of soil based on their type, grading, and geotechnical properties, and some of them, such as swelling clays, cannot be used at all. The types that can be used may have adequate compressive strength, but the soil lacks tensile strength, as well as limited shear strength. As a result, similar to the use of rebar in concrete reinforcement and the invention of reinforced concrete, materials such as geosynthetic materials have been developed and used to compensate for the soil’s lack of tensile strength and improve its other properties, such as drainage capacity, bearing capacity, permeability, and so on.
Geosynthetic materials include geotextiles, geogrids, geonets, geomembranes, geocells, geopipes, and geocomposite, with geogrids used to strengthen and reinforce soil. Previously, reinforced soil referred to the interaction of soil layers with metal belts, in which the tensile forces applied to the soil due to friction between the soil and the metal belt were transferred from the soil to the belt and supported by it. Geogrids are becoming increasingly replaced with metal belts in reinforced soils, for a variety of reasons, including metal belt corrosion in the soil and their higher cost when compared to geogrids.
In this study, we first discuss the various types of geogrids and their characteristics, followed by a description of how to design geogrid-reinforced embankments.
Overview
Geogrids are plastic grids with large holes that are stretched in one or two directions to improve the mechanical qualities of soil. Geogrids alone have at least 25 uses, which can be grouped into two broad categories: reinforcement and separation. Actuality, their application as separators is limited. Geogrids improve soil tensile strength through two main factors: soil friction with geogrids and soil particle locking in geogrid aperture. The factor of soil and geogrid friction is greater in sandy soils, whereas the factor of soil particles locking in geogrid aperture is greater in crushed gravel.
Geogrids are classified into three types: uniaxial, biaxial, and triaxial, and the type used in each project is chosen by the project’s specific characteristics. Figure 1 depicts 3 kinds of geogrids.
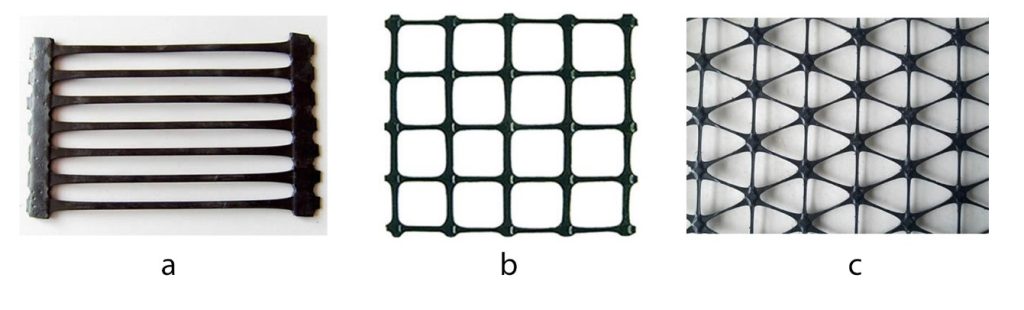
Figure 1: (a) Uniaxial geogrid, (b) Biaxial geogrid, (c) Triaxial geogrid
Polypropylene and high-density polyethylene are the primary materials utilized in the building of high-resistance geogrids. These materials are first formed as very thick plates, which are then punched with a precise pattern to create holes, and finally stretched from one or both sides. Stretching is done at a specified temperature and strain rate to avoid cracks. Figure 2 shows how geogrids are produced.
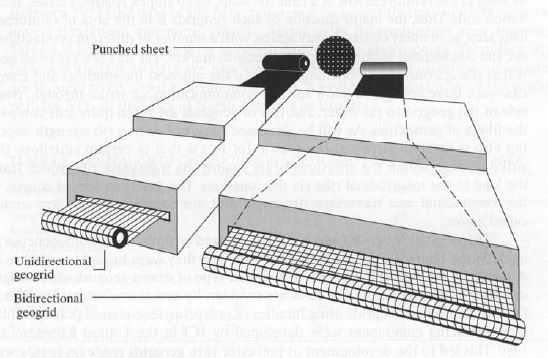
Figure 2: How to Create a Uniaxial and Biaxial Geogrid.
Application of geogrids
- As previously mentioned, geogrids have multiple uses, the most significant of which are listed below:
- Reinforcement of several layers of embankments on the road, airport, and railway.
- Reinforcement of different layers in the slopes and trenches
- Reinforcement of soil layers in reinforced soil walls
- Use as gabion networks along the edges of roads and waterways.
- Reinforcement of different pavement layers such as sub-base, base, and asphalt and fixing defects such as weak sub-base and cracks in the old asphalt surface before coating.
- Separation of gravel layers and road paving layers
- Using geogrids alone for drainage and filtration if the aperture dimensions are small; otherwise, with geotextile.
Considering that the purpose of this research is to investigate and use geogrids in embankments, Figure 3 displays an embankment reinforced with geogrids.
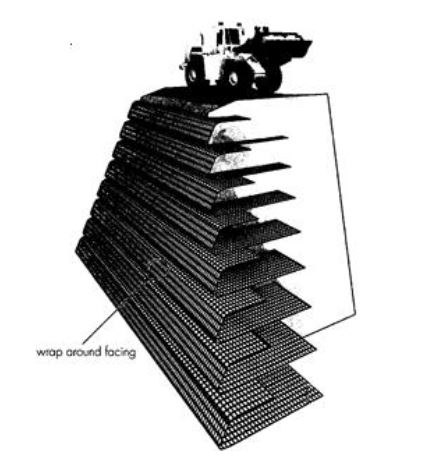
Figure 3: Embankment reinforced with geogrid
Installation of geogrid in embankments
The placement of the reinforcing layer in earthen structures is decided by the project’s technical conditions and the technique of execution. Figure 4 depicts different geogrid reinforcing layer installation patterns in landfills with dense soil.
Figure (4-a) shows that the length of the network is the same to each other, and the vertical distances between them do not change with respect to the embankment’s height. Figure (4-b) shows that the vertical distances between the grids have changed in relation to one another, increasing as they approach the upper range of the soil (the top of the soil). This arrangement is employed when the stresses in the embankment’s lower sections are significantly greater than those in the top sections.
In many cases, heavy soil compacting equipment (such as rollers and other road building vehicles) cannot be moved to the edge of landfills. Therefore items on the landfill’s outside edges are not properly compacted. In other words, the quality of soil compaction will vary depending on where the soil’s outside edge meets its center points. As a result, in such situations, both the embankment body and the corresponding body will be weakened. External components, such as humidity and soil erosion, have a stronger impact on the outer borders and under the shoulder of the road (road sides) than in other areas.Therefore, secondary geogrid networks are used between main networks to increase the stability and durability of the outer edge, prevent the landslip between the reinforcement networks, and compensate for the lack of required soil density in these parts. Auxiliary networks usually have a shorter length than the main geogrid networks. They are spread horizontally between them and at proportional intervals. An example of reinforced embankment along with auxiliary networks is shown in figure (c-4). Here, the length of the main geogrid networks can be equal to each other or different from each other at different heights (Figure 4-d).
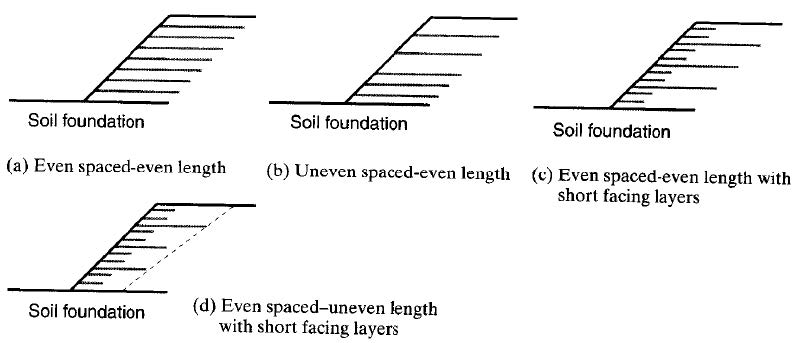
Figure 4: How to Place the Geogrid on an Embankment.
In addition, the geogrid network and the external concrete cover that connects to it have been used in the building of reinforced soil walls. This technology allows you to enhance the slope of reinforced embankment up to 90 degrees. This type of reinforced wall is highly suitable and cost-effective to use in urban areas where land limitations are an important factor. (Figure 5).
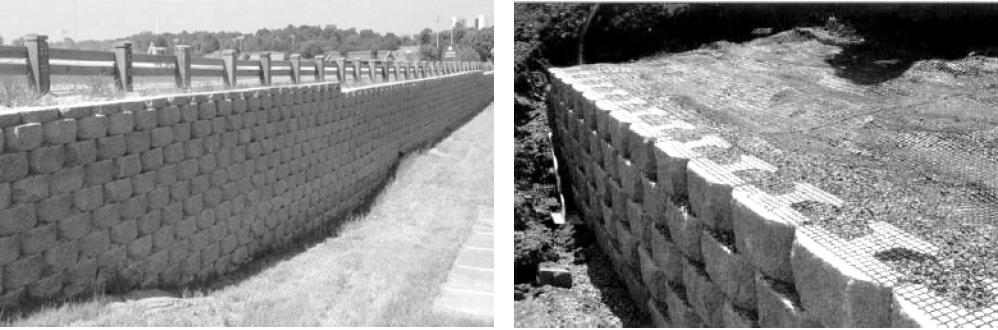
Figure 5: Reinforced soil wall with concrete outer cover
Basics of designing reinforced embankments
Until recently, different approaches for designing geosynthetics in various engineering fields have been provided, including soil reinforcement, layer separation, drainage, filtration, and sealing. Some of these strategies are entirely theoretical, while others combine both theoretical and practical features. In general, the various methods of design described in reliable sources are classified into the following three categories:
- Design based on cost of operation and availability of materials
- Design based on required specifications
- Design based on geosynthetic performance
Full explanations about the above three methods are beyond the scope of this research. However, considering that the performance-based design method is the basis of our design in this research, I introduce this method.
The design concept of geosynthetics based on performance involves the following three steps:
The first step is to identify the most important function of geosynthetics. This means that the most important geosynthetic function to consider should be chosen from among its capabilities. The second stage is to determine the numerical value of the required characteristic with respect to the primary function. Finally, equation 1 is used to calculate the plan’s overall confidence coefficient. In embankment design, geogrid’s most significant function is reinforcement. In addition, the tensile strength of the geogrid is necessary based on its performance.
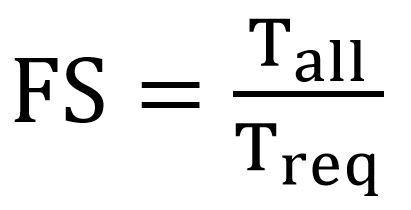
Eq. 1
In this case, Tall, is the tensile strength given by the laboratory analysis, and Treq is the required tensile strength calculated in step 2.
It is important to note that laboratory results usually provide the geogrid’s tensile strength in its ultimate state (Tult), which does not correspond to the actual conditions. As a result, keep in mind that the virtual resistance utilized in the design is lower than the final laboratory resistance (Tult > Tall).
One way to find the allowed strength using the final resistance is to utilize multiple reduction coefficients to modify for laboratory limitations. Equation (2) can be utilized to calculate the allowed tensile strength based on the ultimate tensile strength.
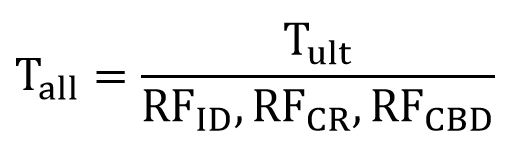
Eq. 2
In this equation, RFID is the reduction factor due to failures during installation, RFCR is the reduction factor due to creep effects during the life of the structure, and RFCBD is the reduction factor due to chemical and biological failures. The values of these coefficients are listed in the table below.
Application type | RFID | RFCR | RFCBD |
---|---|---|---|
Unpaved roads | 1.1-1.6 | 1.5-2.5 | 1.0-1.6 |
Paved roads | 1.2-1.5 | 1.5-2.5 | 1.1-1.7 |
Embankments | 1.1-1.4 | 2.0-3.0 | 1.1-1.5 |
Slopes | 1.1-1.4 | 2.0-3.0 | 1.1-1.5 |
Walls | 1.1-1.4 | 2.0-3.0 | 1.1-1.5 |
Foundations | 1.2-1.5 | 2.0-3.0 | 1.1-1.6 |
Design of reinforced embankments
The limit state theory can be applied to embankments reinforced with geogrids with minor modifications, and the safety factor of embankment against sliding can be calculated. Figure 6 shows the influence and performance of geogrid networks in slope modeling. According to this picture, the existence of reinforcing layers at various levels of the embankment with adequate length adds extra horizontal forces to the set of sliding-resistant forces. In this method, the safety factor of embankment stability improves.
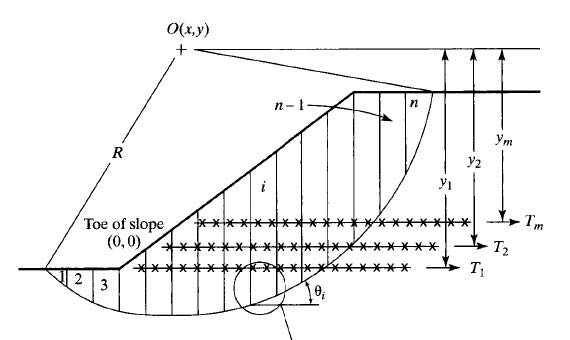
Figure 6: Details of forces acting on the end of a reinforced embankment segment
The effective factors in the creation and formation of tensile resistance forces in geogrid networks are, firstly, the mobilized friction force between the soil and the geogrid network, and secondly, the displacement amount created in the embankment parts.
In other words, if any of the above variables are missing from the mechanism, the geogrid is unable to function in the embankment. The safety factor of embankment stability against sliding is calculated using equation (3).
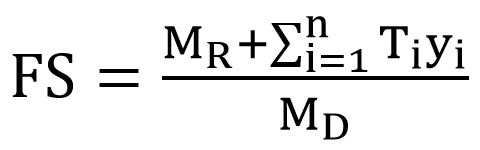
Eq. 3
In this equation, FS is the slope sliding safety factor, MR is the momentum resistant to sliding due to the soil’s shear resistance, and MD is the momentum of the driving forces caused by the weight component of the slices, seepage pressure, earthquake force, and the effects of other dead and live loads on the embankment. Ti indicates the allowable tensile strength of geogrid networks, yi represents the resistant momentum arm, and m represents the number of geogrid layers.
The study and design of reinforced slopes are based on the slope’s stability in order to avoid sliding and define a minimum saftey ffactor. Because of many failure mechanisms and geometric details of the sliding surfaces, these processes can take a long time to complete. As a result, researchers have attempted to give simpler analysis and design approaches by investigating the primary failure processes in reinforced slopes based on tests and laboratory studies. The table below lists the names of a few researchers and the details of their approach in the field of reinforced embankment design.
Method | The geometric form of the sliding surface | Pore water pressure | Slope angle | Reinforced length |
---|---|---|---|---|
Ingold | Limited slope | Not considered | 30-80 | Not considered |
Murray | Two-part wedge | Considered | 10-40 | Not considered |
Jewell | Two-part wedge | Considered | 30-80 | Can be calculated |
Leshchinsky | Spiral logarithm | Not considered | 15-90 | Not considered |
Schneider & Holtz | Two-part wedge | Considered | 0-40 | Not considered |
Reugger | Circular | Not considered | 30-90 | Can be calculated |
Schmertmann | Two-part wedge | Not considered | 30-80 | Can be calculated |
Considering that the previously mentioned method, in addition to being difficult to calculate, does not provide information regarding geogrid position and length. Schmertmann’s design method is described below.
Schmertmann’s design method
Schmertman’s approach, which uses wedge limit equilibrium analysis, is used to design reinforced embankments (one- and two-part). Schmertman presented a reasonably basic way by presenting design diagrams based on a set of assumptions. Soil is granular, soil’s characteristics are uniform, and at the highest point of the slope, the ground surface is considered flat; the pore water pressure is zero; and the soil is stable under the embankment. Figure 7 shows the geometric parameters of Schmertman’s theoretical embankment.
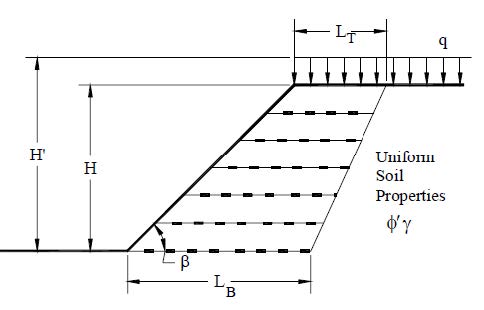
Figure 7: Schmertmann’s design method
To utilize this method, first of all, according to the safety factor chosen for the overall design, reduce the friction angle of the embankment materials using equation 4.
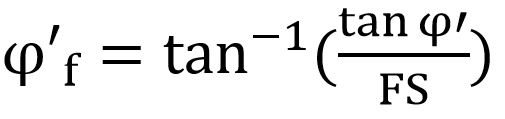
Eq. 4
The reduced friction angle is represented by φ’f, whereas φ’ represents the friction angle of fine soil materials. It should be emphasized that the defined minimal safety factor was determined based on the embankment’s importance and use, the quality of the materials employed, and the project’s execution conditions. However, this value is normally at least 1.5.
The height of the embankment is subsequently modified using equation 5 according to the surcharged load on the embankment.
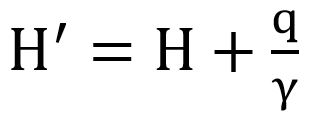
Eq. 5
In this equation, H is the height of the embankment, q is the intensity of the uniform surcharge on the embankment, and γ is the specific weight of the embankment materials.
Then, using equation 6, the value of the maximum tensile force is calculated.

Eq. 6
In this regard, k is the equivalent pressure coefficient of the embankment, which can be obtained from Figure 8, considering the slope of the embankment and the reduced friction angle of the materials.
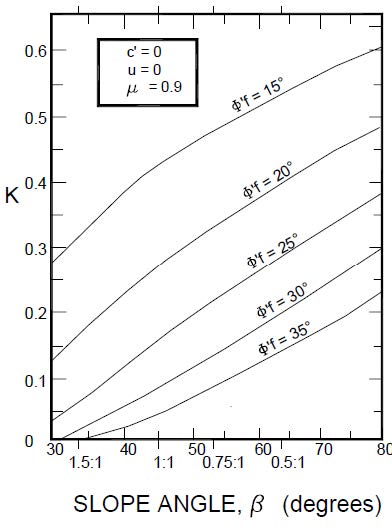
Figure 8: Design diagram for calculating the equivalent pressure coefficient of the embankment
Given that the geogrid employed at each embankment level varies, the maximum tensile stress estimated from equation 6 for the upper third of the embankment is one-sixth of the maximum tensile force. This value is lowered to one-third of the maximum tensile force for the middle third and half of the maximum tensile force for the bottom third of the embankment. The number of geogrid layers is then determined using equation 7.
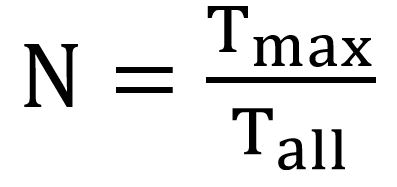
Eq. 7
In this equation, N is the number of geogrid layers, Tmax is the maximum tensile force in the embankment at each height, and Tall is the allowable tensile force measured using equation 2. Also, equation 8 is used to calculate the distance between geogrids.
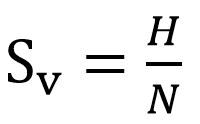
Eq. 8
In this equation, Sv is the distance of geogrids from each other. The suggested distances of the main reinforcing layers in embankments are given in the table below.
Geogrid location | Vertical distance between layers (cm) |
---|---|
For all embankment points | At least 20 to 30 |
the bottom of the embankment | Maximum 60 to 120 |
In the upper part of the embankment | Maximum 180 |
If the distance between the two main geogrid layers exceeds 30 cm, additional layers should be placed between them.
Finally, we utilize Figure 9 to determine the geogrid lengths at the embankment’s top (Lt)and bottom (Lb).
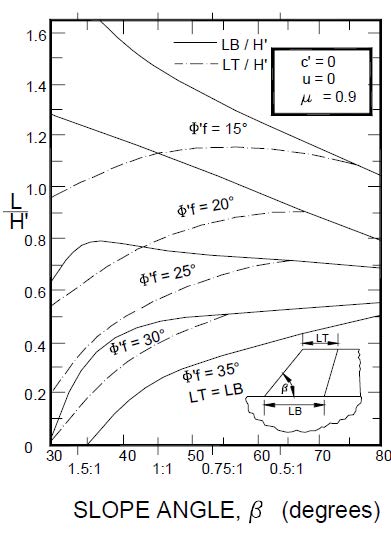
Figure 9 – Design diagram for determining the length of geogrid layers
Conclusion
To reduce the issues associated with unreinforced embankments, certain reinforcing materials or elements such as geogrids must be used. Geogrid-reinforced soil requires compliance to specific technical specifications regarding the type and granularity of the soil, the thickness of the layers, the manner and amount of necessary compaction, geogrid maintenance and installation, and other implementation issues, all of which necessitate the use of experienced and knowledgeable engineers. It is obvious that failing to follow the technical specifications during geogrid implementation is a waste of time and money, and in this case, it is preferable to use traditional methods of implementing retaining walls or methods that are more likely to meet with the technical requirements during implementation and have the technical expertise of their implementation.
Sources
Schmertmann, G. T., Chouery-Curtis, V. E., Johnson, R. D., & Bonaparte, R. (1987). Design charts for geogrids-reinforced soil slope. In Proceedings of the Geosynthetics ’87 Conference (pp. 108-120). IFAI.
0 Comments